In the realm of cellular respiration, oxidative phosphorylation plays a crucial role as the final step in the process. This energy-producing mechanism occurs within the mitochondria and is closely linked to the electron transport chain. Through a series of redox reactions, electrons are transferred from electron carriers such as NADH and FADH2 to oxygen, resulting in the formation of adenosine triphosphate (ATP), the primary energy currency of the cell.
For readers delving into the oxidative phosphorylation and interested in broader biological topics, our lipids and animal cell pages serve as insightful references.
What is Oxidative Phosphorylation?
Oxidative phosphorylation, also known as electron transport-linked phosphorylation or terminal oxidation, is a metabolic pathway in which cells utilize enzymes to oxidize nutrients. By doing so, they release chemical energy, which is then used to produce ATP. This process occurs predominantly within the mitochondria of eukaryotic organisms and is essential for their survival and function. Oxidative phosphorylation is so pervasive because it generates more energy compared to alternative fermentation processes like anaerobic glycolysis.
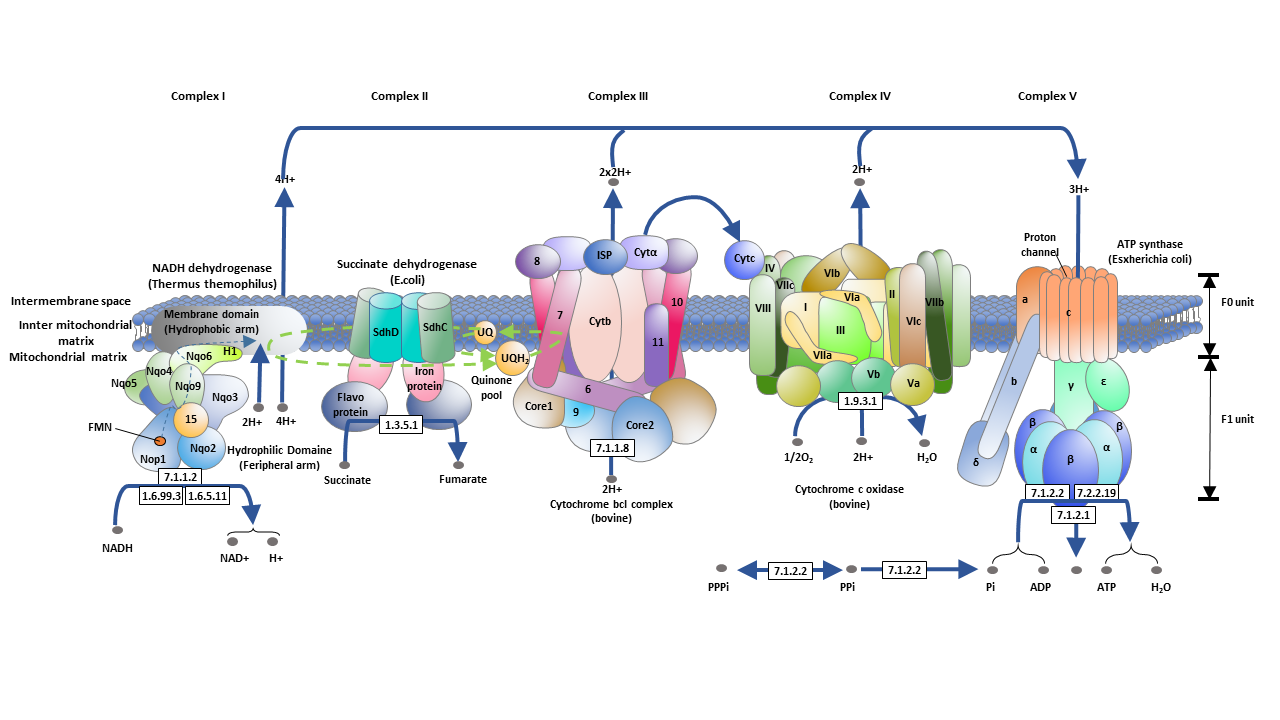
Oxidative Phosphorylation Steps
The major steps of oxidative phosphorylation in mitochondria can be summarized as follows:
Delivery of Electrons by NADH and FADH2
During cellular respiration, reduced NADH and FADH2 molecules transfer their high-energy electrons to molecules near the beginning of the electron transport chain. As a result, NADH and FADH2 get oxidized to NAD+ and FAD, respectively. The oxidized forms of these electron carriers are then utilized in other steps of cellular respiration.
Electron Transport and Proton Pumping
In the electron transport chain, the transferred electrons move from a higher energy level to a lower energy level, releasing energy in the process. Some of this energy is used to transport protons (H+) from the matrix to the intermembrane space, establishing an electrochemical gradient.
Splitting of Oxygen to form Water
The electrons, after being transported through the electron transport chain, are transferred to oxygen molecules. This process involves the splitting of oxygen into two parts, with one part combining with hydrogen ions (H+) to form water.
ATP Synthesis
The H+ ions, which have accumulated in the intermembrane space, flow back into the matrix through an enzyme called ATP synthase. This controlled flow of protons through ATP synthase facilitates the synthesis of ATP.
Chemiosmosis
Oxidative phosphorylation involves the coupling and interrelation of exergonic processes (electron transport chain) and endergonic processes (ATP synthesis). The energy released during the exergonic electron transport chain is transmitted to ATP synthase through the movement of proteins, a process known as chemiosmosis. This ensures the efficient production of ATP.
Electron Transport Chain
The electron transport chain is a series of protein complexes that facilitate the transfer of electrons from electron donors (such as NADH) to electron acceptors (such as oxygen). In eukaryotes, the electron transport chain is located in the inner mitochondrial membrane. It consists of five main protein complexes, each with specific roles in the electron transfer process. The complexes are:
- NADH-coenzyme Q oxidoreductase (complex I)
- Succinate-Q oxidoreductase (complex II)
- Electron transfer flavoprotein-Q oxidoreductase
- Q-cytochrome c oxidoreductase (complex III)
- Cytochrome c oxidase (complex IV)
These complexes contain various cofactors, including iron-sulfur clusters, flavin adenine dinucleotide (FAD), and heme groups. Through a series of redox reactions, these cofactors facilitate the movement of electrons along the electron transport chain.
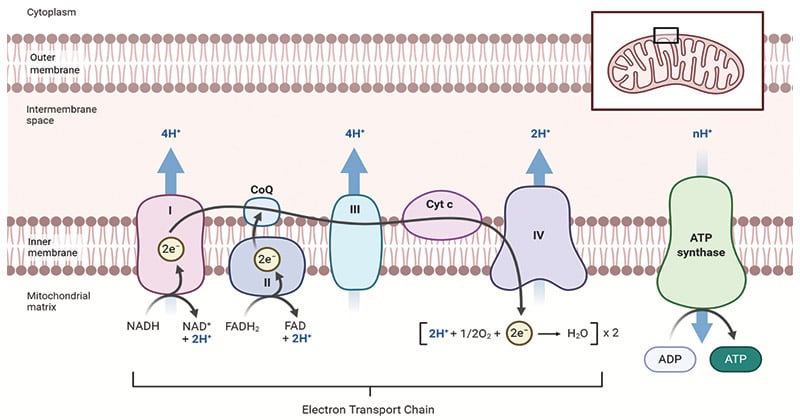
Electron and Proton Transfer Molecules
In the process of oxidative phosphorylation, electrons are transferred between various molecules known as electron and proton transfer molecules. These molecules play a crucial role in facilitating the flow of electrons through the electron transport chain. Some examples of these molecules include:
- Cytochrome c: This water-soluble electron transfer protein carries electrons between complexes III and IV in eukaryotes.
- Coenzyme Q10 (Q): This lipid-soluble electron carrier transfers both electrons and protons along the electron transport chain. It undergoes reduction and oxidation reactions, converting between its oxidized (Q) and reduced (QH2) forms.
- Flavin cofactors: These include flavin mononucleotide (FMN) and flavin adenine dinucleotide (FAD). They accept and donate electrons during redox reactions within the protein complexes of the electron transport chain.
- Iron–sulfur clusters: These clusters, such as [2Fe-2S] and [4Fe-4S], are involved in electron transfer processes. They facilitate the movement of electrons between different electron carriers.
These transfer molecules allow for the efficient and controlled flow of electrons through the electron transport chain, enabling the generation of ATP.
Eukaryotic Electron Transport Chains
Eukaryotic organisms, including humans, have complex electron transport chains that consist of multiple protein complexes. These complexes work together to transfer electrons and establish an electrochemical gradient. Let’s explore some of these complexes:
NADH-coenzyme Q oxidoreductase (complex I)
Complex I, also known as NADH-Q oxidoreductase, is the first protein in the electron transport chain. It is a large enzyme with multiple subunits and catalyzes the oxidation of NADH and the reduction of coenzyme Q10. As electrons flow through complex I, protons are pumped from the mitochondrial matrix to the intermembrane space, contributing to the electrochemical gradient.
Succinate-Q oxidoreductase (complex II)
Complex II, or succinate-Q oxidoreductase, is unique as it is involved in both the citric acid cycle and the electron transport chain. It oxidizes succinate to fumarate and reduces coenzyme Q10. Unlike other complexes, complex II does not transport protons across the membrane and does not contribute to the proton gradient.
Electron transfer flavoprotein-Q oxidoreductase
This enzyme, also known as ETF-Q oxidoreductase, accepts electrons from electron-transferring flavoprotein in the mitochondrial matrix. It transfers these electrons to coenzyme Q10, contributing to the electron transport chain. ETF-Q oxidoreductase plays a role in the metabolism of fatty acids, amino acids, and choline.
Q-cytochrome c oxidoreductase (complex III)
Complex III, or cytochrome bc1 complex, transfers electrons from coenzyme Q10 to cytochrome c. This complex contains cytochromes, iron-sulfur clusters, and coenzyme Q10. As electrons pass through complex III, protons are pumped from the matrix to the intermembrane space, contributing to the proton gradient.
Cytochrome c oxidase (complex IV)
Complex IV is the final complex in the electron transport chain. It is responsible for transferring electrons from cytochrome c to oxygen, reducing oxygen to water. Complex IV contains multiple subunits, heme groups, and metal ion cofactors. As electrons flow through complex IV, protons are pumped across the membrane, further contributing to the proton gradient.
Alternative Reductases and Oxidases
In addition to the main complexes, eukaryotes can have alternative reductases and oxidases that play a role in electron transport. These enzymes, such as alternative NADH oxidases and the alternative oxidase, allow for flexibility in electron transfer pathways and can provide protection against oxidative stress.
Organization of Complexes
Recent research suggests that the protein complexes of the electron transport chain may form higher-order structures called supercomplexes or “respirasomes.” These supercomplexes facilitate the efficient transfer of substrates and channeling of electrons between the different complexes. However, the exact nature and significance of these supercomplexes are still under investigation.
Oxidative Phosphorylation Products and Equation
The primary product of oxidative phosphorylation is adenosine triphosphate (ATP), which serves as the primary energy currency of the cell. Through the process of chemiosmosis, the flow of protons through ATP synthase drives the synthesis of ATP from adenosine diphosphate (ADP) and inorganic phosphate (Pi). For every molecule of NADH oxidized, approximately 2.5 molecules of ATP are generated, while the oxidation of each succinate molecule yields approximately 1.5 ATP molecules.
The equation for oxidative phosphorylation can be summarized as follows:
NADH + H+ + 1/2O2 + ADP + Pi → NAD+ + H2O + ATP
This equation represents the overall process of oxidative phosphorylation, where NADH and H+ (protons) from the electron transport chain combine with oxygen and ADP to produce NAD+, water, and ATP.
Diagram of Oxidative Phosphorylation in the Mitochondria
The diagram above illustrates the process of oxidative phosphorylation within the mitochondria. It highlights the electron transport chain, the movement of protons across the inner mitochondrial membrane, and the synthesis of ATP through ATP synthase.

Solved Examples on Oxidative Phosphorylation
Example 1:
A cell undergoes oxidative phosphorylation, and for every molecule of NADH oxidized, 2.5 molecules of ATP are generated. If the cell oxidizes 10 molecules of NADH, how many ATP molecules will be produced?
Solution: Number of ATP molecules = Number of NADH molecules × ATP produced per NADH molecule Number of ATP molecules = 10 × 2.5 Number of ATP molecules = 25 Therefore, 25 ATP molecules will be produced.
Example 2:
During oxidative phosphorylation, how many molecules of water are formed when 8 molecules of oxygen are reduced?
Solution: Number of water molecules = Number of oxygen molecules × Number of water molecules formed per oxygen molecule Number of water molecules = 8 × 1/2 Number of water molecules = 4 Therefore, 4 molecules of water are formed.
Example 3:
What is the role of ATP synthase in oxidative phosphorylation?
Solution: ATP synthase is an enzyme located in the inner mitochondrial membrane. Its role is to facilitate the synthesis of ATP from ADP and inorganic phosphate (Pi). ATP synthase uses the flow of protons (H+) through it to drive the phosphorylation of ADP, resulting in the production of ATP. This process is known as chemiosmosis.
How Kunduz Can Help You Learn Oxidative Phosphorylation?
At Kunduz, we understand the importance of mastering complex biological processes such as oxidative phosphorylation. That’s why we offer a range of resources and tools to enhance your learning experience. Our comprehensive study materials, interactive lectures, and practice exams are designed to help you grasp the concepts of oxidative phosphorylation and excel in your biology studies.
Whether you’re a high school student preparing for exams or a college student pursuing a degree in the life sciences, Kunduz is here to support you every step of the way. With our user-friendly platform and affordable pricing, learning about oxidative phosphorylation has never been easier or more accessible. Join Kunduz today and unlock your full potential in the fascinating world of biology.